HELICOPTER ARTICLES: written by various authors :
Aerodynamics of Autorotation
source: HelicoptersOnly.Com
During powered flight, the rotor drag is overcome with engine power. When the engine fails, or is deliberately disengaged from the rotor system, some other force must be used to sustain rotor RPM so controlled flight can be continued to the ground. This force is generated by adjusting the collective pitch to allow a controlled descent. Airflow during helicopter descent provides the energy to overcome blade drag and turn the rotor. When the helicopter is descending in this manner, it is said to be in a state of autorotation. In effect the pilot gives up altitude at a controlled rate in return for energy to turn the rotor at an RPM which provides aircraft control. Stated another way, the helicopter has potential energy by virtue of its altitude. As altitude decreases, potential energy is converted to kinetic energy and stored in the turning rotor. The pilot uses this kinetic energy to cushion the touchdown when near the ground
Most autorotations are performed with forward airspeed. For simplicity, the following aerodynamic explanation is based on a vertical autorotative descent (no forward airspeed) in still air. Under these conditions, the forces that cause the blades to turn are similar for all blades regardless of their position in the plane of rotation. Dissymmetry of lift resulting from helicopter airspeed is therefore not a factor, but will be discussed later.
During vertical autorotation, the rotor disk is divided into three regions:
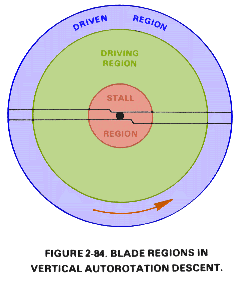
- The driven region, also called the propeller region, is nearest to the blade tips and normally consists of about 30 percent of the radius. The total aerodynamic force in this region is inclined slightly behind the rotating axis. This results in a drag force which tends to slow the rotation fo the blade.
- The driving region or autorotative region, normally lies between about 25 to 70 percent of the blade radius. Total aerodynamic force in this region is inclined slightly forward of the axis of rotation. This inclination supplies thrust which tends to accelerate the rotation of the blade
- The stall region includes the inboard 25 percent of the blade radius. It operates above the stall angle of attack and causes drag which tends to slow the rotation of the blade
The following figure shows three blade sections that illustrate force vectors in the driven region "A", a region of equilibrium "B" and the driving region "C":
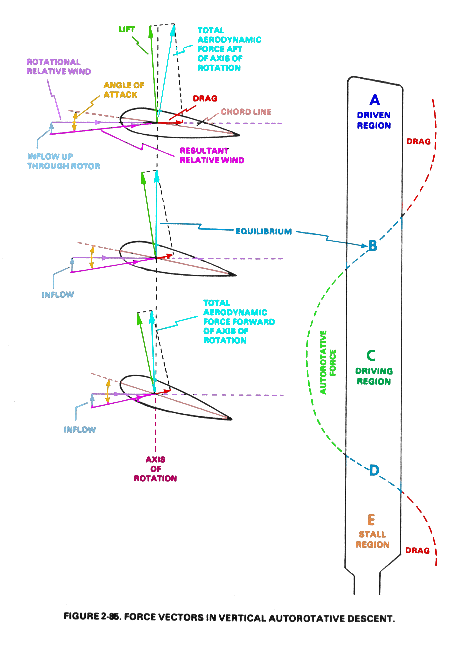
The force vectors are different in each region, because the rotational relative wind is slower near the blade root and increases continually toward the blade tip. When the inflow up through the rotor combines with rotational relative wind, it produces different combinations of aerodynamic force at every point along the blade.
In the driven region, the total aerodynamic force acts behind the axis of rotation, resulting in an overall dragging force. This area produces lift but it also opposes rotation and continually tends to decelerate the blade. The size of this region varies with blade pitch setting, rate of descent, and rotor RPM. When the pilot takes action to change autorotative RPM, blade pitch, or rate of descent, he is in effect changing the size of the driven region in relation to the other regions.
Between the driven region and the driving region is a point of equilibrium. At this point on the blade, total aerodynamic force is aligned with the axis of rotation. Lift and drag are produced, but the total effect produces neither acceleration nor deceleration of the rotor RPM. Point "D" is also an area of equilibrium in regard to thrust and drag.
Area "C" is the driving region of the blade and produces the forces needed to turn the blades during autorotation. Total aerodynamic force in the driving region is inclined forward of the axis of rotation and produces a continual acceleration force. Driving region size varies with blade pitch setting, rate of descent and rotor RPM. The pilot controls the size of this region in relation to the driven and stall regions in order to adjust autorotative RPM. For example, if the collective pitch stick is raised, the pitch angle will increase in all regions. This causes the point of equilibrium "B" to move toward the blade tip, decreasing the size of the driven region. The entire driving region also moves toward the blade tip. The stall region becomes larger and the total blade drag is increased, causing RPM decrease.
A constant rotor RPM is achieved by adjusting the collective pitch control so blade acceleration forces from the driving region are balanced with the deceleration forces from the driven and stall regions.
MORE ON THIS AND MANY OTHER HELICOPTER RELATED TRAINING TOPICS AT : HELCICOPTERSONLY.COM
|